Airplanes, Cars”What‘s the Difference?
What you need to know, on the road and in the air
What's the difference between car (or motorcycle) engines and aircraft engines? It's a perennial question with a series of stock answers, none of which is sufficient.
It's as obvious as asking the difference between cars and airplanes, but it's as technical as explaining the differences between car pistons and airplane pistons. The quick answer is, "They do different things." The longer answer is more interesting, so let's get right into it.
Demands differ. It takes 12 to 15 horses to run a small car 60 to 70 mph (cruise speed), and the car's peak horsepower is 10 times that. Cars never run at full power for more than a few seconds; airplanes may use 100% all the way to cruise altitude. The car spends a lot of its life at a 10% power setting; an airplane at 70% to 80%. Also, part of the drag the airplane engine overcomes is due to a wing's requirement to produce lift (tires produce all the lift a car needs), so the engine isn't just moving the airplane through the air, it's also holding it up. With a helicopter hovering OGE (out of ground effect), this is more obvious, though the principle is the same as in an airplane.
Duty cycles differ. Two thousand car hours typically represent between 70,000 and 100,000 miles, about half the car's expected life. With a piston-airplane engine, 2,000 hours is about all we expect. On the other hand, the car will run out that mileage in five to seven years; a GA airplane will last 40. Airplane engines are used infrequently, hard and for relatively short times, all of which promote additional stress and wear.
Operating environments are different. While it's no picnic under the hood of a car, it's worse inside a cowling. Particularly with air-cooled aircraft engines, temperature stresses and rates of change can be enormous. A 100-degree day on the ground can be below zero in mere minutes at altitude. Rain can shock unshielded air-cooled cylinders and heads. Manual operation of aircraft cooling systems (e.g., cowl flaps) means that these systems are operated suboptimally at best and incorrectly at worst, introducing additional stress.
Operators are different. Finally, we see a factor in favor of the aircraft engine! In general, pilots are better attuned to their engines than are car drivers. Offsetting this advantage, though, is the fact that pilots face more demands from their engines, which require more attention and are fussier about fuel type and grade, throttle and mixture settings (to say nothing of prop settings!), and temperature management, all of which are irrelevant or automatic in modern cars.
Maintenance is different. Modern cars don't need "tune ups." Unleaded gasoline, modern electronics, improved metallurgy, and constant design and material enhancements have coalesced into today's modern engines that require only periodic fluid changes to stay healthy above 100,000 miles. Airplane engines have few of those advantages, but they do receive a professional look at least once a year---something car engines don't get (and usually don't require).
![]() |
![]() |
A 301-cubic-inch, 500 hp V10 (top) in a BMW M5, water-cooled with a redline at 8,250 rpm. A Continental IO-550N (bottom) in a Cirrus SR22. At a 550-cubic-inch displacement, it turns out 310 hp at 2,700 rpm. |
The consequences of failure are different. When a car's engine fails, you can simply pull over; when an airplane's engine fails, you have to land. Pulling over at an unplanned spot is much easier than landing at an unplanned spot (particularly if it's dark). Extra safety margins are expected and required in aircraft.
So, why? A lot of the differences have to do with the fact that it's not economically feasible to make changes to existing aircraft engine designs. While a car-engine redesign gets its expenses amortized over many hundreds of thousands (or even millions) of units in a relatively short time, aircraft engines are sold by the dozen. Any change has a greater impact on average cost.
Costs of changes, too, are different. Assuming that all changes are going to be improvements (a huge and not entirely reliable assumption), an auto-engine improvement is largely an internal affair. The manufacturer does its own evaluation, design, testing and appraisal. Expensive, certainly, but that's the price of progress. An aviation engine builder has all those expenses, plus additional, significant considerations.
An aviation-engine manufacturer needs to explain and justify its changes through the "certification" process with the FAA and various CAAs. Not only are the processes, tests and documentation expensive, but also the time involved can't be overestimated. These regulators are bureaucracies, accountable to essentially no one, so they work on their own schedules.
There's also the fact that current designs work. They have proven themselves for the past 70 years or so. We know how to operate them and how to maintain them; we know what weak spots to monitor. They may not be perfect (and they aren't), but we can live with their needs, much like an old spouse.
So, why change? We change because we have to and because we want to. As fuels change, we need to adapt. As energy costs rise, we want more economical operation. As metallurgy improves, we can save weight, repair time and money by employing better materials.
What can we learn from cars? A hundred years ago, even 60, "aircraft technology" meant "superior." The Tucker, the most-advanced "production" car of its time, used a 300 hp water-cooled Franklin aero engine right after WWII. Recent history, though, has seen technology going the other way, toward automotive-style metallurgy, higher-rpm engines and gearboxes, automotive-style electronic engine controls, auto-style fuel injection, and adoption of auto fuels, including unleaded gasoline and diesel.
A decade and a half ago, Bob Pond shook up the Reno race crowd with his high-revving race car engines and a lightweight airframe (in his Unlimited Class Pond Racer). Frank Thielert has recently brought automotive technology to aviation, in the form of his relatively tiny (121-cubic-inch, two-liter) diesels, now in the Diamond DA42 and several other aircraft under STC. Rotax, for nearly 20 years, has offered a geared, 1.1-liter, auto-gas-burning (but still carbureted) engine that powers many of the new LSAs. Lycoming and Teledyne Continental Motors (TCM) have models that feature fuel injection, modernized ignition and one-lever operation.
What do we have to do, and what do we want to do? It won't be long before 100LL disappears. (We've been hearing it for 30 years, so it must be true.) Lead is poison; we don't want to use it when we don't have to. We still have to use it because it's the only practical way to boost octane (required by high-compression gasoline engines), and because the majority of aircraft piston engines need that octane to keep running. Though 80/87 worked for a lot of peashooters, sales volume was too low to allow its continued production, so we went to "low-lead" in the 1970s. (Note that 100-octane "low-lead" avgas contains two grams of tetraethyl lead per U.S. gallon, half that of aviation 80/87 and 100/130, but some 18 times that of the automotive premium of the 1970s.)
Eric Tucker, who knows the Rotax engines inside-out, said that auto gas (which is specified for the Rotax engines) and automotive-style (motorcycle-style, actually) oils make a good match, because all the components---engine, fuel, gearbox and oil---are designed to work together. When legislators (who are usually lawyers, not engineers) mandated the removal of lead from fuels, they had no idea of the secondary effects they were setting in motion (or they paid no attention). In addition to the octane-boosting effects of lead, Tucker said, "The designer relied on lead in the fuel to help reduce valve seat and valve wear, but now the old fleet is stuck because it must have the lead, or face expensive changes. Auto-engine oil has wear inhibitors to reduce wear, but it has created wear issues of another kind, such as camshaft and [flat-tappet] lifter issues."
Tucker offered some advice for leaded-gas users: "Lead, combined with moisture, makes a chemical acid which can burn the bearings and leave pitting in aluminum surfaces. Long periods of nonuse, typical for an aircraft, cause many issues with lead contamination, one of them being the chemical actions that take place. The best practice is to change the oil before storage (draining the acids with the old oil). When people don't do this, they keep the overhaulers busy."
In the interest of fuel efficiency, we'll need to have more-efficient engines. That means not only fuel-saving improvements (electronic engine controls, fuel injection, tighter tolerances, better cooling, improved lubricants), but also smaller and lighter engines. Metallurgy will provide many of the breakthroughs---lighter pistons and rods allow lighter cranks and cases, for instance---but additional fuel-flow, combustion and exhaust-design improvements are still necessary.
We also need lighter propellers that can produce good thrust from smaller diameters (reducing gyroscopic, straight-line and angular momentum, reducing the amplitudes of harmonics and running quieter at any given rpm while affording sufficient ground clearance, which in turn lowers airframe weight). Smaller engines can reduce frontal area or form drag; liquid-cooled engines often afford design flexibility in internal drag reduction that's only sometimes offset by component weight and system complexity. Smaller engines also help reduce airframe weight: Attach points and hardware, engine mounts, etc., can all be smaller and lighter.
What's being done, now? We see constant improvement in existing engine technology: Aftermarket and OEM-direct shops such as Unison and K&N offer largely bolt-on incremental improvements through their STCs. New lubricants and coolants, including semi- and full synthetics, improve performance and component life. Detail improvements (such as improved metallurgy cylinders, new valve and head designs, roller lifters, plastic plenums) reduce weight or wear. Further along the evolutionary scale, increased use of turbochargers helps produce more power from any given package; FADEC (full-authority digital engine control) systems outperform even the most experienced and attentive pilots. Additional movement is promised by geared engines, diesels and compound-turbo-supercharged technology.
Ian Walsh, VP and General Manager at Lycoming (also a Six Sigma black belt), noted that the obvious costs can be reduced, and not only through higher production numbers. "Automotive manufacturers have become best in class when it comes to lean and cost-out efforts," says Walsh, "because they've pioneered lean implementation, Kaizen ’continuous improvement,' Six Sigma methodologies and supply-base rationalization and transformation. Aviation is learning the same techniques and process improvements to make innovation more affordable."
The piston engine will be around for a long time in its present configuration, if for no other reasons than that the installed base is so great and the costs of replacement are so high. New-generation induction, engine control and flow management (both internal and external---combustion and cooling) improvements will become more economical and widespread. Diesel technology, accepted worldwide (and grudgingly in the States), will become more popular as avgas becomes ever more dear. Smaller engines, geared engines, lower-power engines---all are in the near future. In other words, we'll see progress on all fronts!except in bureaucracy.
Walsh concluded, "We should be excited that aviation engines are becoming more technologically advanced, economical and will undoubtedly become more affordable as automotive thinking plays a more influential role. Competition, in the final analysis, is the best creator of all!"
Go, Speed Racer |
High-performance driving parallels flying more than you might imagine |
By Jeff Berlin |
The head-up display in my peripheral vision is reading 127 mph. Wow, I'm really flying. Pilots hearing such a statement might think, "Head-up display? Must be a jet," and in most cases, they would be right, but not this time. Actually, I'm not in an airplane at all. I'm at the two-day BMW M School performance driving course at the California Motor Speedway, and I've got the pedal to the metal in a $100,000 BMW M6, unleashing as many of the 500 horses of its growling V10 as I can. From where I sit, careening down a curvy asphalt ribbon, as I prepare to stand on the brake for all I'm worth to slow for turn one, I'm in a dichotomy of pure luxury and unbridled performance---think autobahn on Sunday afternoon meets Le Mans.
BMW defines performance driving (per-for-mance dri-ving; verb, transitive) as "The act of extracting the highest level of performance from an automobile by its driver under any circumstances," and on these two days, not only did I learn a helluva lot about controlling a car at the razor-sharp edges of its envelope, but I also learned how similar performance driving and flying really are. This similarity was readily apparent from before my classmates and I fired up our M6s, M5s and Z4 M Coupes. Once we all signed our lives away on lengthy waivers with lots of fine print and got fitted for our 1950s retro-style helmets to channel Ricky Bobby, we congregated in the Speedway's driver meeting room for a presentation by Jim Millard, a BMW Performance Center instructor, about the dynamics of high-performance driving, which covered one of the most important issues to any active pilot---decision making. According to Millard, "One of the school's main focuses is to reinforce good decision making behind the wheel while building confidence and maximizing fun. We want drivers to exercise good judgment while building their awareness of the driver/car relationship and while learning how to comprehend the car's handling---what the car is telling you." Besides exercising proper judgment while careening at breakneck speed from a straight into a switchback, or using similar discretion as an aviator to ensure maximum flight safety, there's another element at play that directly translates from flying to driving and back again---physics. The kinetic and dynamic forces that act upon a car, especially when taken closer to its limits, are very similar to those experienced by pilots in aircraft. During his presentation, Millard mentioned that we'll be exercising these cars in their three axes: vertical, longitudinal and in the transverse, or to pilots, yaw. (Wait, you mean that cars also have three axes? Who knew?) During practice, we'll each be learning how to better feel the car and understand what it's telling us. Then we'll take those individual elements reinforced during drills and string them together on the road course. Millard then described how a car's center of gravity directly affects its performance on the track. Center of gravity relates directly to a car's vertical axis as it accelerates and brakes. BMW M cars are balanced with 50/50 front/rear weight distribution for optimum handling. Using throttle inputs to smoothly manipulate this vertical axis allows the driver to maximize tire traction and transfer weight forward or aft, to the tires that need to do more work at any given moment. In an aircraft, center of gravity is much more critical, and like a car during acceleration, a rearward center of gravity will facilitate a bit more speed since the horizontal stabilizer will need to create less negative lift. The car's longitudinal axis, what we pilots call roll, directly correlates to aviation a bit less, since cars and airplanes effect turns differently, with lateral turning forces affecting each differently. In the transverse, however, there's a direct correlation that can be demonstrated in a car by oversteering in a turn, which will cause what most call a fishtail. In an airplane, overshooting the turn from base to final and trying to rudder it around might be a good example of, perhaps, airplane oversteer. But there are other, more intuitive lessons I also learned during my high-revving weekend at the speedway. One of the most notable is getting into the habit of driving as smoothly as possible. Millard mentioned that the best race car drivers are always the smoothest, and while I've no desire to become a race car driver (though I wouldn't mind enrolling next in BMW's Advanced M School), our passengers and equipment, car or plane, will all appreciate driving or flying as smoothly as possible. And like in auto racing, smoothness also pays dividends at the highest levels of sport aviation. According to air show star and three-time national aerobatic champion Patty Wagstaff, by smoothly finessing her 350 hp Extra 300S through her air show routine and by keeping the plane coordinated and, in her words, happy, she'll wring more performance and dissipate less energy than if she were to be too aggressive with control inputs. And because she's flying in front of millions each air show season, flying her show smoothly also makes it look good to the crowds. "Look at how smoothly Bob Hoover flew," she mentioned. Indeed. Another parity between high-performance driving and flying, which Millard and his instructors drilled into our skulls from the get go: Keep your eyes up. How many times have we heard from flight instructors to keep our eyes outside, especially as more and more pilots transition to potentially mesmerizing glass cockpits? Millard taught us this weekend not only to keep our eyes up, but also to look far down the track, to find our turn points as early as possible and to visualize our lines around the track. What a terrific habit to get into when flying, especially in the airport environment. When do I want to turn base or final? Where's my other traffic? Where do I want to touch down? In the car, where you're looking is where your hands will take the car. Looking far into a turn or far down the road, even when off the track and on surface roads or the highway, will pay off in increased safety and reaction times. And speaking of reaction, at the beginning of the school I was a bit hesitant and tentative with control input and really getting on the brake. But after a couple days of track work, decelerating numerous times from 130 to 35 to make my turn and not run off onto the infield got me over any hesitation of applying whatever full control inputs might be necessary to guide the car through the course. The parity here: Many pilots also are loath to apply full control deflection in aircraft. I had an inkling as I sat in the driver meeting room that first morning that flying and performance driving might bisect at one point or another, but never did I expect them to be as connected as they are. After my stint in BMW's M School, not only did I become a better driver, but I also became a better pilot. For more, log on to www.bmwusa.com. |
Lycoming To The Max! |
Flying with Bill Stein |
Engine operations during normal flight may be straightforward, but what goes on when an engine is pushed to its limits? We asked air show pilot Bill Stein (www.billsteinairshows.com) to analyze performance of his Zivko Edge 540's Lycoming IO-540 as he spins and tumbles his way through his high-energy aerobatic sequence.
Throughout the remainder of the sequence, my engine encounters a predicable set of challenges. An inverted flat spin will slow the engine down to 2,400 rpm, and oil temp and CHT rise due to lack of airflow through the cowl. My spiraling tower concludes with an upright flat spin, and the engine bogs down again, but there's no drop in oil pressure. Many people assume that the end-over-end tumbles are the most violent figure I fly, but inside the cockpit it's relatively smooth and I rarely exceed -2 G's. However, tumbles do exert a huge side load on the prop and the airframe, and the engine moves around significantly. (I once had to relocate a cowl attach screw after the engine was pushed so far to the left that the starter ring teeth had not only eaten up the screw, but had also gotten close enough to the cowl to destroy the nut plate as well.) Of any figure, I'm told that snap rolls put the biggest load on the crank, due to the rapid, simultaneous pitch change and yaw motion. When the stick is yanked back and the rudder is stomped on, the spinning propeller (attached to the engine crank) gyroscopically resists these position changes. Snap rolls are always a part of my sequence, but they're also great fillers, especially on vertical lines, so I usually sprinkle a dozen or so inside and outside snaps throughout a show. Most pilots are concerned with shock cooling; I'm one of them, except when I'm flying at a show. Toward the end of my sequence, I fly an inverted low pass, push for an outside half loop to a couple of revolutions of a centrifuge and then park the airplane midair for a harrier. It was during this that I obtained my highest CHT readings to date. In a harrier, the airplane hangs on the prop at a very high deck angle, and it feels like I'm trying to balance while standing on top of a basketball. With a good setup, the airplane can stay parked in one spot for a long time---so long, in fact, that most of my attention is devoted to CHT, and I'll exit the harrier as the temps reach 430 to 450 degrees. Only two figures remain in my sequence after the harrier, so I often land with a very hot engine. During practice, I have the luxury of slowly cooling engine temperatures before entering the pattern, but air shows mean entertainment, so in front of the crowds I must get on the ground as soon as possible to keep the show rolling. It's this kind of engine abuse that explains my engine rebuild and overhaul schedule of every 500 to 700 hours. During a performance, my airplane and I both take a lot of punishment. But that's only the tip of the iceberg---because I treat every practice like it's an air show, this scenario repeats at least 300 times per year. I'm glad that I fly the best aerobatic airplane built, equipped with the best aerobatic engine from Lycoming and the best ignition system from Unison. I'm often asked what the riskiest thing is that I do in the Edge, and my reply is flying cross-country from show to show. Every time I look down at the Sierra Nevada range or the Rockies and all I see are trees and cliffs with no place to land, I think about the trust and confidence that I have in my airplane and especially my engine. |
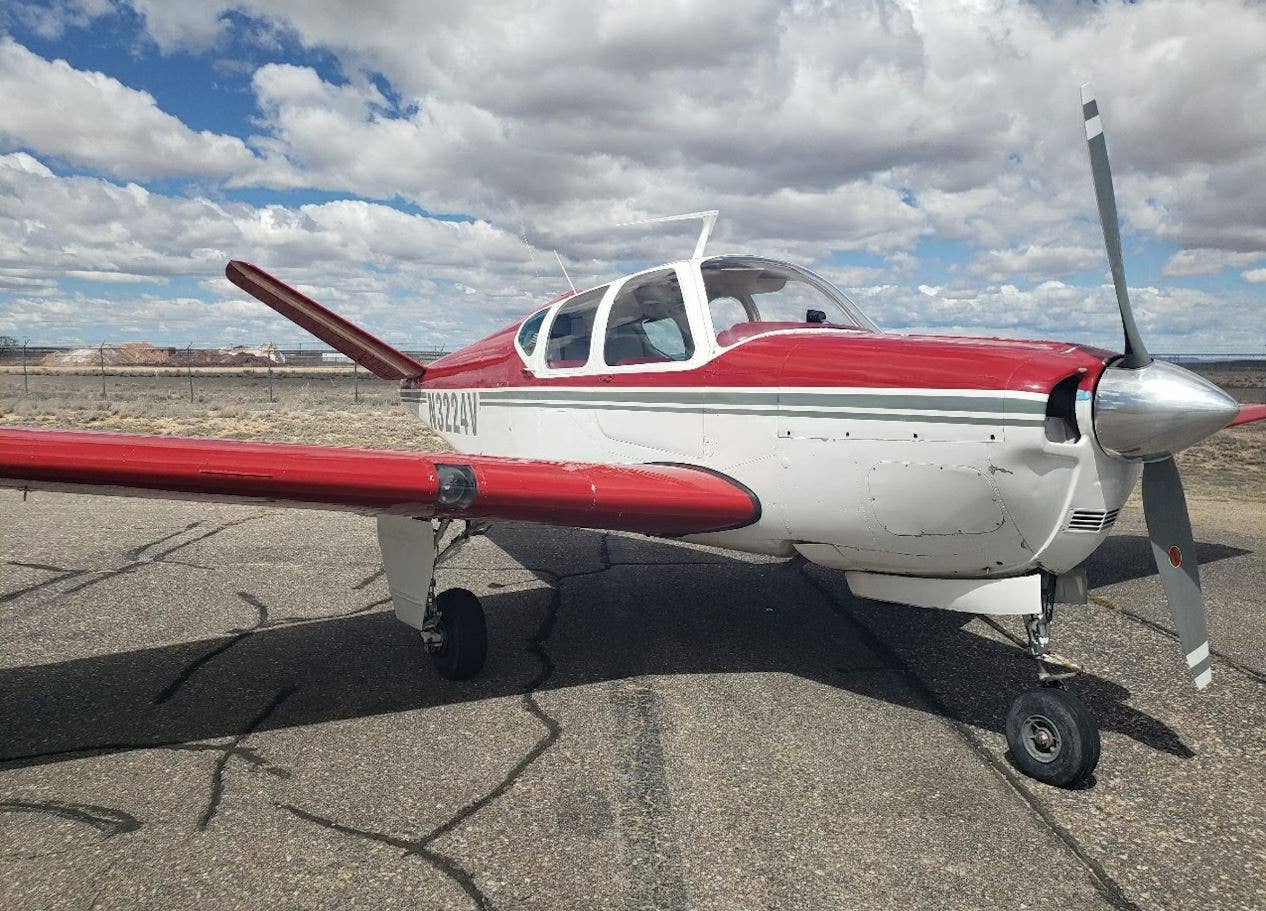
Subscribe to Our Newsletter
Get the latest Plane & Pilot Magazine stories delivered directly to your inbox